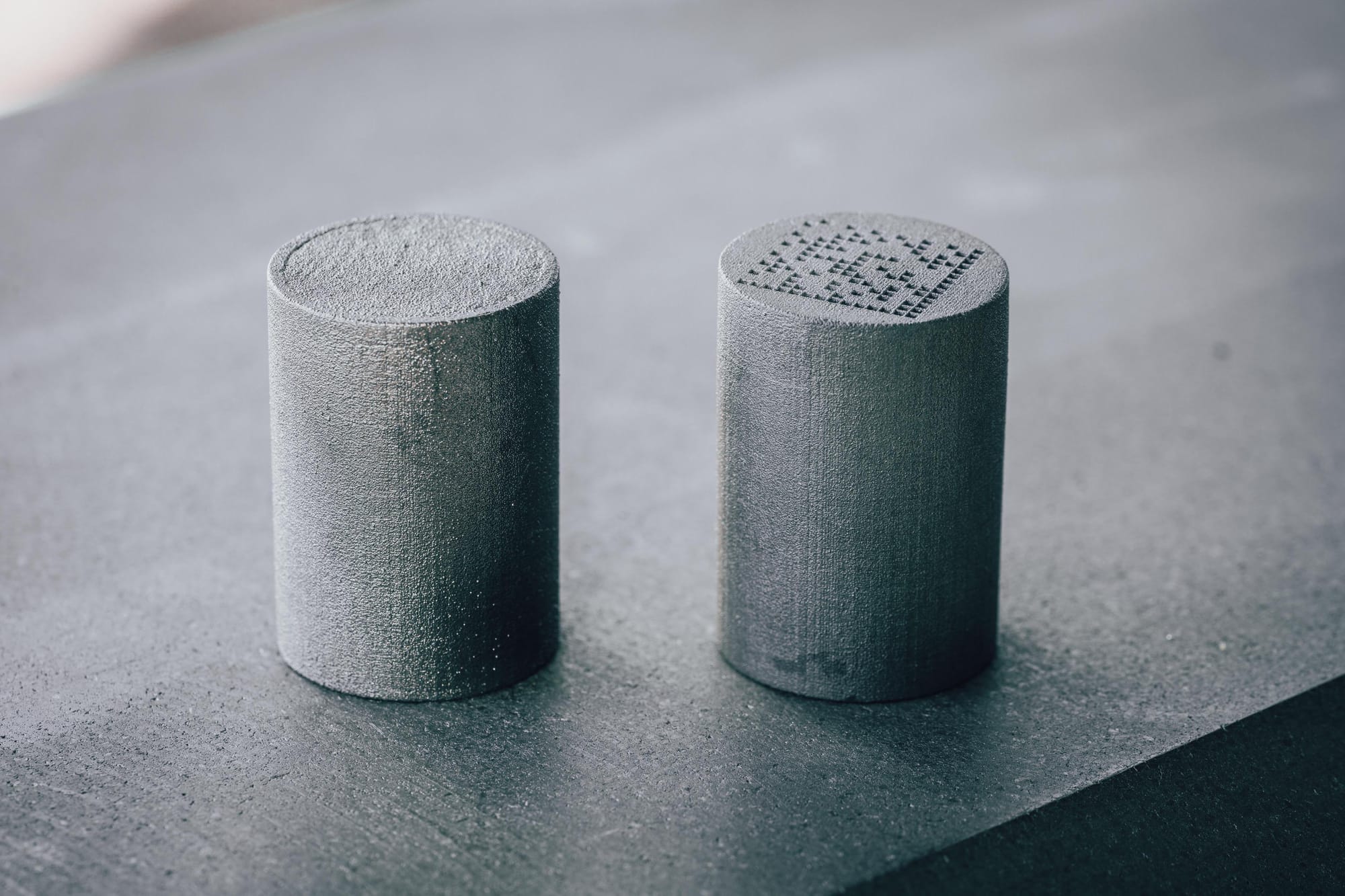
Nuclear Fuels
The conventional UO2/Zircaloy fuel and TRISO based fuels are introduced below on the basis of how they address or exacerbate the hazards present in a nuclear reactor. Compared to conventional fuel, TRISO aims to contain and limit the fission product risk at a much smaller scale with higher performance materials thereby limiting the state space and growth of periphery coping systems. TRISO’s hazard reduction goes far beyond that of conventional fuel. Its potential to allow for simplified reactor systems may outweigh its higher fabrication costs.
Conventional Fuel
Conventional nuclear fuel is UO2 oxide pellets inside a Zircaloy tube, called a cladding. As the fuel is used, the UO2 expands and crumbles into pieces and pressurizes the Zircalloy tube. The fission products, gases, and solids, are released into the tube, creating a high pressure (>40MPa) in the tube. Just beyond the coolant operating conditions of roughly 400 °C, the Zircaloy tube will experience excessive corrosion over its 4-6 year lifetime. By 700-900 °C Zircaloy tube can burst and by 1000 °C it can generate significant amount of hydrogen gas in an exothermic reaction with water. In any of these cases, if the UO2 fuel operates at greater than 800 °C, fission gases are released into the coolant. The hydrogen generation from Zircaloy, if left unmitigated and its underlying critical safety functions for a reactor will likely lead to a hydrogen explosion or fire. The containment will then hold the pressure of the accident and withstand impacts of hydrogen explosions, fires, and elevated temperatures. If helium is utilized as the coolant, then the limiting temperature for conventional fuel is closer to 800 °C. Even at 1000 °C, very limited fission gas inventory is released from UO2 pellets.
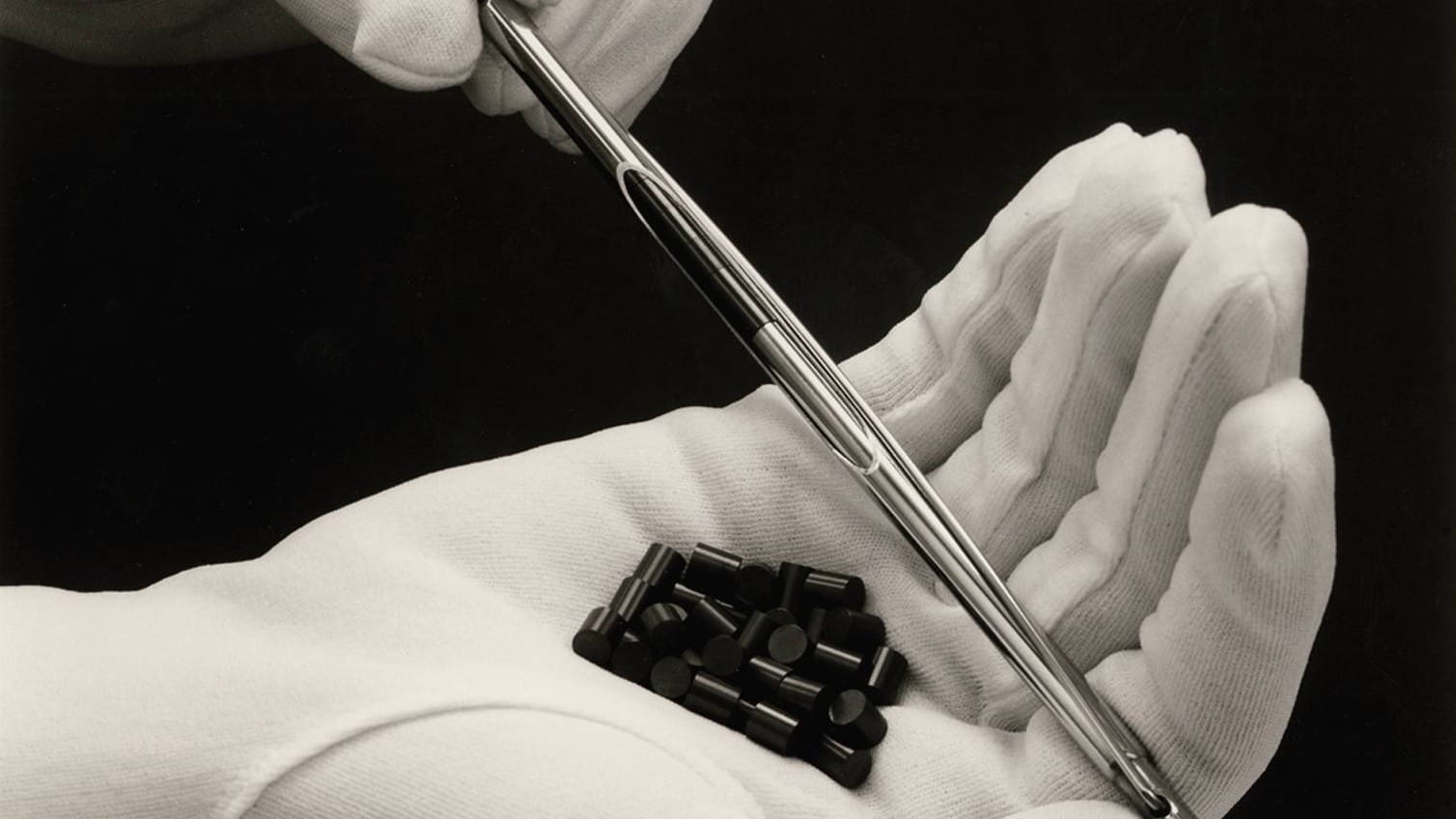
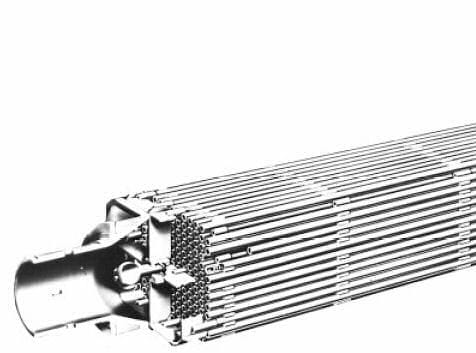
Conventional nuclear fuel pellets (left) and Zircalloy clad fuel assemblies (right). From NEA (2020), Nuclear fuel pellets - image 2, OECD Publishing, Paris;
TRISO/FCM Fuel
Originally conceived in 1957 [1] during the British Dragon program, TRistructural ISOtropic (TRISO) particle fuel consists of fissile Uranium or Thorium fuel kernels measuring 250 to 600 μm across and coated with layers of Pyrolytic Carbon and Silicon Carbide (sometimes ZrC for NTP applications). TRISO particles are the fuel spheres with ceramic layers, while the matrix is the ceramic material that surroundings the TRISO particles to form a consolidated fuel pellet, usually a cylinder, measuring about 1-2 cm in diameter. The matrix is traditionally graphite, but use of SiC has also been explored.
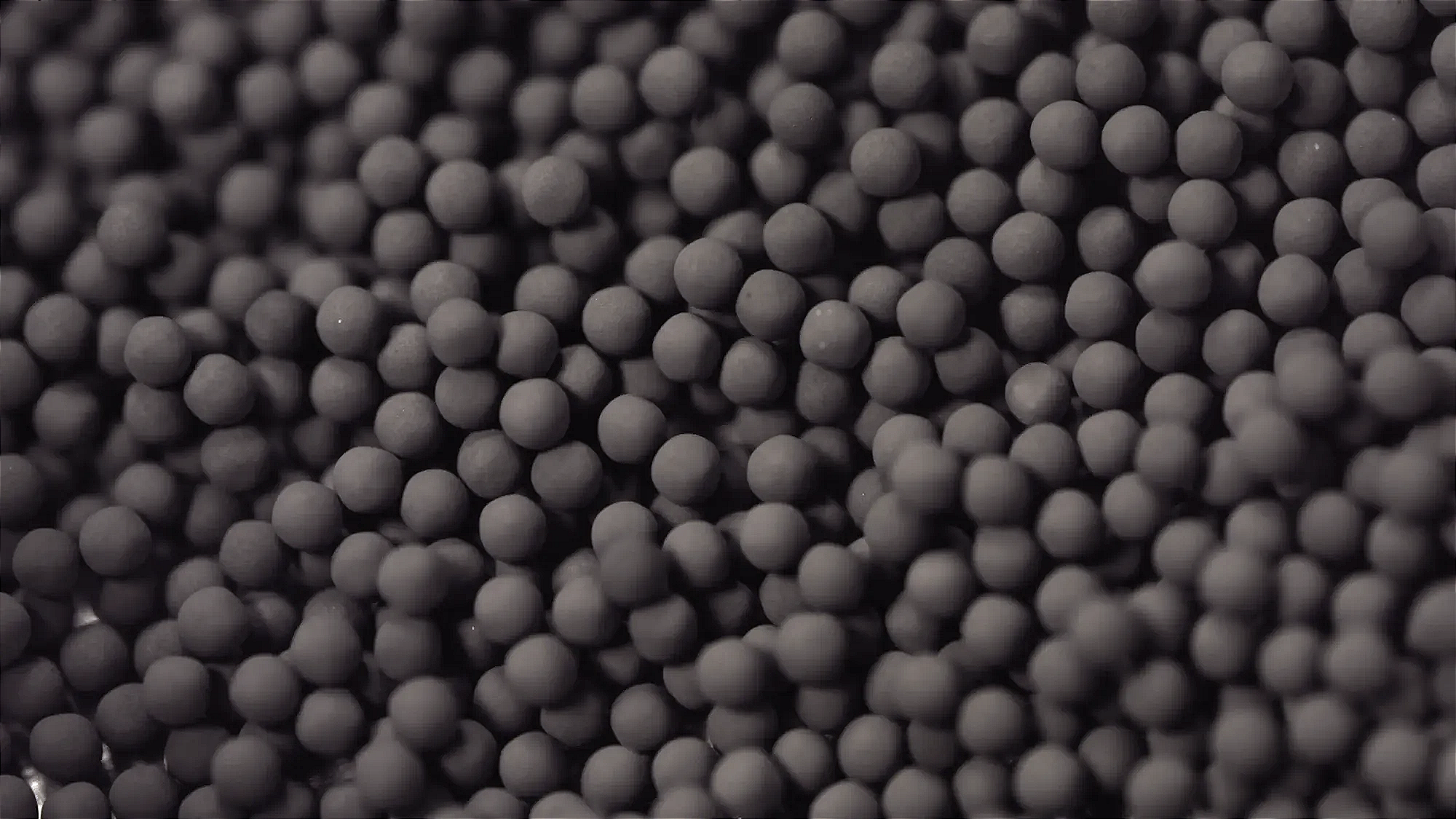
The driving idea behind TRISO particle fuel is to give each 250 to 600 μm sized piece of nuclear fuel its own containment and pressure vessel to enhance the fuel’s ability to contain fission products at very high temperatures and neutron bombardment. The physical basis for miniaturizing the pressure vessel is twofold: fuel subdivision and pressure vessel enhancement.
First, subdividing the nuclear fuel by four to five orders of magnitude significantly reduces the risk of single point failures in the pressure vessel or fuel cladding. Ordinarily, if a single failure in the reactor’s main steel pressure vessel or one or few fuel cladding is significant enough, it can lead to a radioactivity release that requires plant shutdown and costly cleanup. With TRISO, particles fail at rates of 10^-5 during operating conditions, and when they occur, lead to a small radiation release into the fuel matrix where it can be stopped to a degree. With TRISO, fission product retention is achieved at the millimeter scale and by high performance ceramics which limits the accessible state space and the growth of periphery systems at a larger scale. Addressing problems at the smallest feasible scale reduces the required overhead containment effort before they have a chance to grow in size, area, and rate. In fact, U.S. NRC has accepted he idea of a “functional” containment and for HTGRs, it is expected to not require expensive pressure retaining containments. With conventional fuel, much effort must be taken to prevent their escape. Subdivision of fuel into particles is even believed to provide blast resistance as the particle may be small and durable enough to remain intact during explosive fractures like a direct missile hit. Indeed, with this reasoning in mind, the US Department of Defense began funding development of TRISO fueled reactors for forward bases in early 2019.[2]
The second advantage to miniaturizing the pressure vessel is the ability to enhance pressure vessel performance through use of thin layered ceramics in a mass manufactured, seamless, spherical design. Fabrication of millions of particle pressure vessels can be highly standardized and controlled in a mass manufacturing environment with defect rates of 1 in 100,000.[3] Crucially, the millimeter scale vessel allows the use of highly pure brittle ceramics made through CVD techniques that maintain high strength and stability under high temperature and irradiation and have dual use as low reactivity fission product barriers. Normal reactor pressure vessels are made one at a time and have to be cylindrical, with various seaming and joining techniques, multi cm wall thickness, and cannot use brittle materials. As the pressure vessel size is reduced, the more efficient spherical geometry can be adopted, and the required wall thickness drops dramatically to the point that a 35 μm SiC layer can indefinitely contain gas pressures in excess of 200 MPa.[4] This compares to the main reactor steel pressure vessel which may go as high as 15MPa or the Zircalloy cladding which can have pressures up to 50-100 MPa in high temperature conditions. As the nuclear fuel fissions, it continues to accumulate fission product gases and increases the gas pressure that must be contained. If the pressure vessel can handle higher pressures, the fuel can be burned more extensively and safely, which means more efficient use of fuel.
The attractiveness of TRISO-matrix fuel is derived from its ceramics' high thermal conductivity, high fission product retention, and superior irradiation and corrosion resistance across operating, accident, and storage conditions. This translates into better efficiency and safety for current civilian reactors (operation at higher temperatures with higher safety margins) as well as upcoming micro reactors, gas cooled reactors, and molten salt cooled reactors. More extreme performance applications enabled by TRISO-matrix type concepts include nuclear thermal propulsion (NTP) for space, VHTR, Subterrene tunneling, and nuclear ramjets.
TRISO in Graphite
TRISO is traditionally packaged into graphite pellets. These pellets are formed through a high pressure and high temperature process with various organic binders. The process allows for only simple pellet shapes and can lead to overstressing of the particles, though this can be controlled. Although the TRISO particle is an excellent fission product retention device, some fission products like Ag and Ce can leak into the coolant. More particles will fail as temperatures are elevated in the core during extreme accident conditions. The graphite pellets or pebbles will tend to undergo irradiation induced swelling and property changes and sometimes crack, impacting the heat transfer from fuel to coolant and complicating spent fuel handling with extra processing steps before permanent storage.
TRISO in SiC Matrix – FCM Fuel
TRISO particles must be packaged into a fuel form or pellet, and SiC encapsulation, also known as Fully Ceramic Micro-encapsulated Fuel (FCM)[5] or Fuel-in-Fiber [6], offers a series of benefits over graphite including higher fission product retention, greater tolerance to air/steam ingress, and potentially higher burnup capability. The most significant benefit to SiC encapsulation is that the additive process used in its manufacture can achieve around 65% packing fraction with little to no stress on the TRISO particles during manufacturing, although this can ostensibly be accomplished with graphite as well. This process also gives design freedoms for near-arbitrary fuel form factors such as annular fuel and other highly specified shapes that can vary 3-dimensionally across the core.[7] This allows a reactor to operate at higher power and with greater energy content without increasing temperatures in the fuel. Other benefits include reduced dimensional changes of the pellets, reduced thermal property changes of the pellets over time and radiation damage. SiC ends up swelling instead of shrinking and its swelling saturates after 1 DPA.[8] Finally, the SiC or graphite encapsulation creates a ready-for-storage form factor that is simpler to handle than traditional crumbling fuel pellets and a step above simple graphite encapsulated TRISO.
SiC-encapsulation may enhance the resilience of TRISO particles to higher temperatures and irradiations allowing for more extensive burnups, higher power densities, and extreme accident tolerance. Higher burnups allow for a reduction in the long-lived fission products, reducing the spent fuel’s radioactivity lifetime to thousands of years rather than hundreds of thousands.
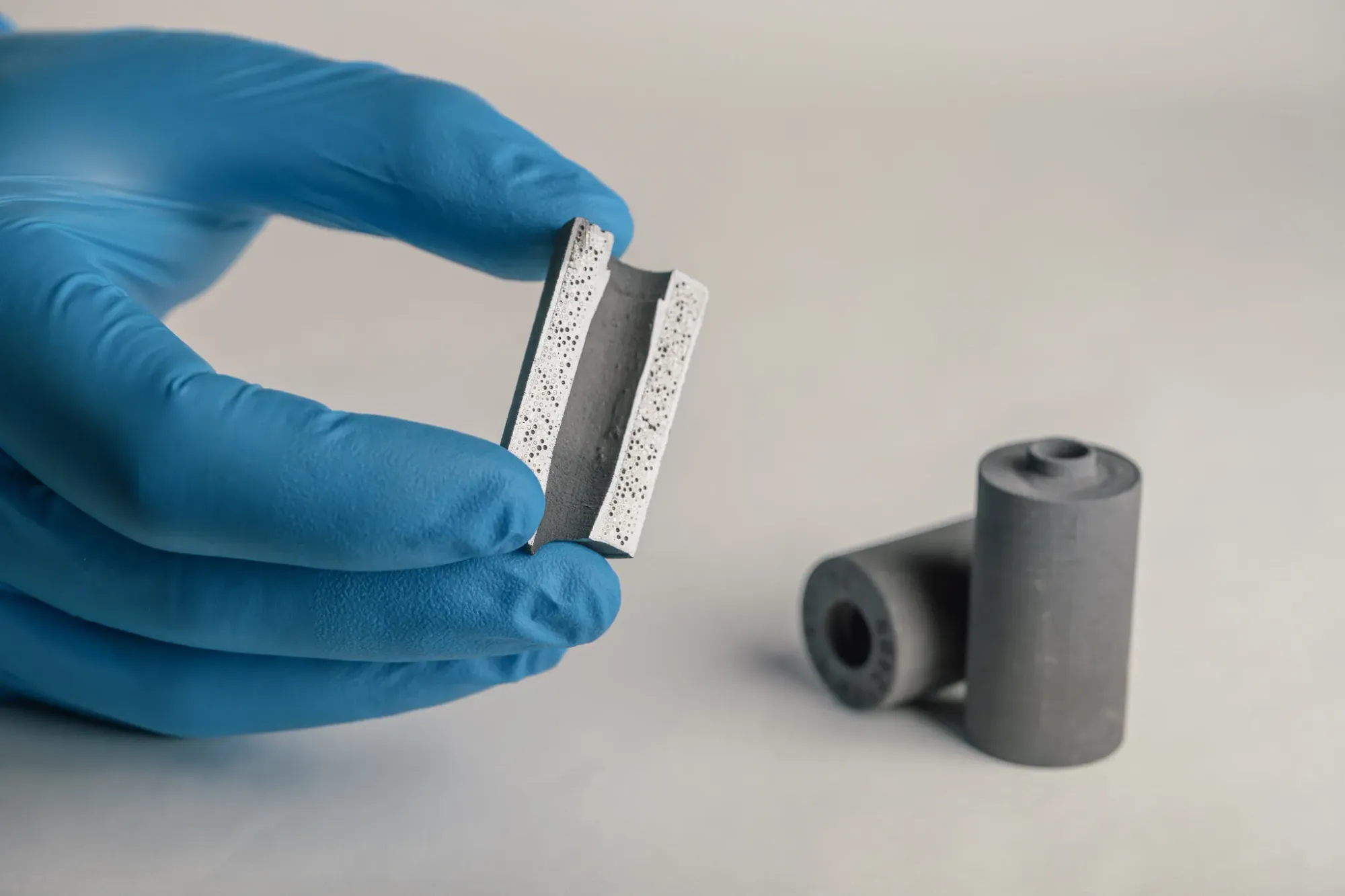
Fuel Enrichment
HALEU greater than 5% enrichment does not exist in the US outside the military complex, and significant quantities are unlikely to be available for demonstrations before 2030, let alone commercial rollouts. The capability to use lower enrichment fuel unlocks several benefits with a cascade of knock-on advantages. The lower enrichment fuel improves the risk profile for technology demonstration and deployment. Supply chains do not have to be created from scratch and favorable regulations and facility requirements are allowed for lower enrichment fuel, which will directly impact fuel fabrication and reactor manufacturing costs. Finally, lower fuel enrichment reduces strategic value and proliferation risk because it takes more effort to further enrich to weapons capable grades.[9]

[1] K. Verfondern, H. Nabielek, and J. M. Kendall, “Coated Particle Fuel for HTGCR.”
[2] “PELE PROGRAM PHASE I.”
[3] Petti et al., Triso-Coated Particle Fuel Performance.
[4] Hales et al., “Multidimensional Multiphysics Simulation of TRISO Particle Fuel.”
[5] Snead et al., “Fully Ceramic Microencapsulated Fuels: A Transformational Technology for Present and next Generation Reactors-Properties and Fabrication of FCM Fuel.”
[6] Hiscox and Shirvan, “Reactor Physics Analysis of a New Accident Tolerant Fuel Called Fuel-in-Fibers.”
[7] Terrani et al., “Architecture and Properties of TCR Fuel Form.”
[8] Snead et al., “Handbook of SiC Properties for Fuel Performance Modeling.”
[9] Glaser, “About the Enrichment Limit for Research Reactor Conversion: Why 20%?”