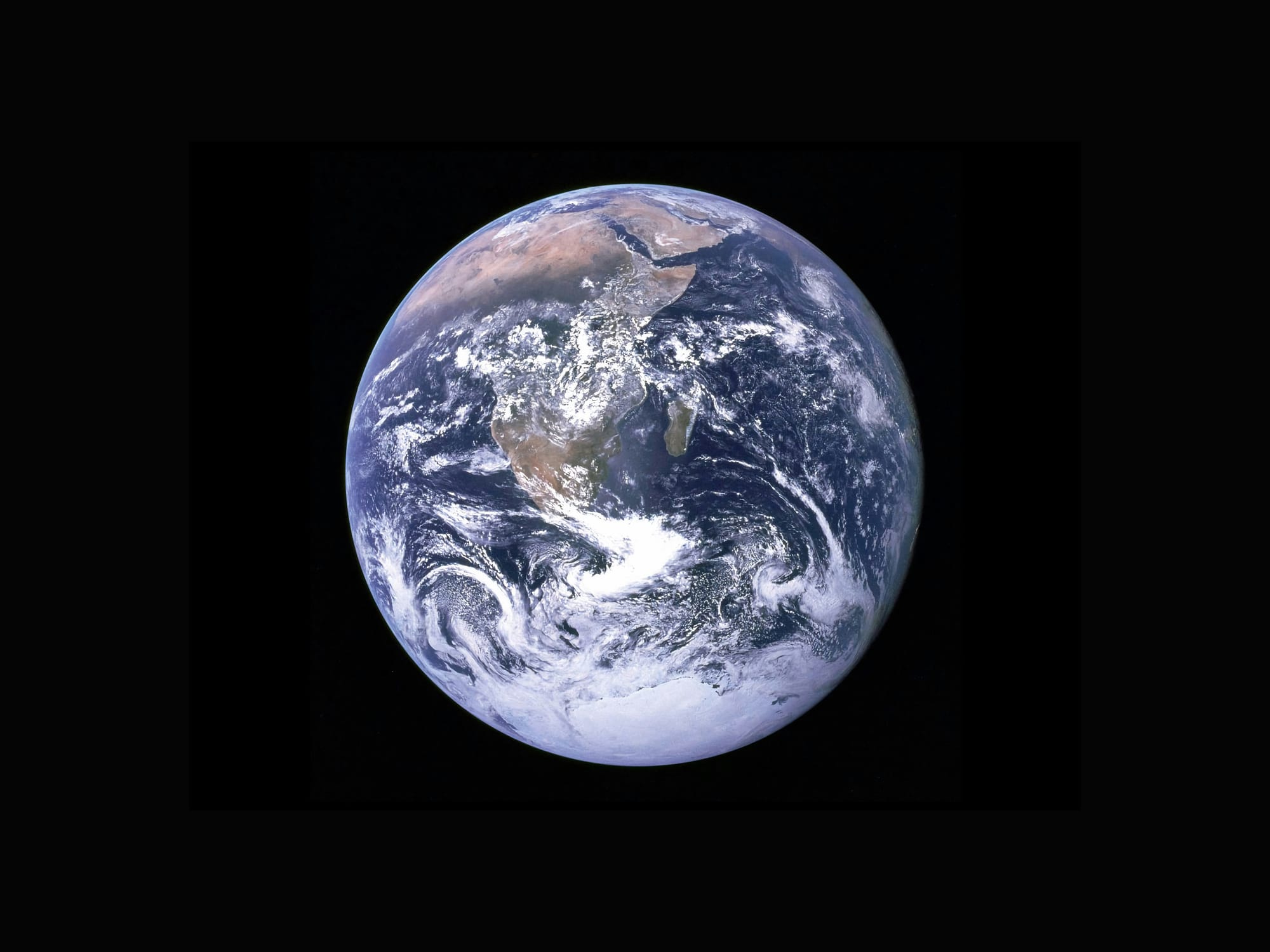
Risk Reduction
Approach for Widescale Adoption
The risk related to nuclear fission is first described and it is argued that aversion to nuclear at the local level is not as unfounded as the nuclear community would make it out to be, considering the alternatives. It is shown that widescale democratic adoption can only be achieved through a different approach to risk reduction. Instead of reducing risk through reduced probabilities and militaristic operations, risk can be reduced by lowering consequences primarily through the use of passive safety mechanisms and inherently safe design characteristics that consider the hazards surrounding the fission products. This was the predominant approach to nuclear safety in the 1990s and gave rise to passive safe designs such as AP1000. In the 1990s both government and public support were low for nuclear energy. Since only the Vogtle units have come online in the last 30 years, it is still not clear, if such a strategy will be ever commercially viable. Nevertheless, this is the direction for nuclear design behind this thesis and many recent SMR and micro reactor demonstration and commercialization efforts, that push passive and inherent safety to the extreme, considering designs where risk can be evaluated deterministically.
Energetic Driving Force Creates Consequence from Fission Products
Nuclear reactors produce radioactive fission products, roughly a third of the periodic table, and these radioactive isotopes pose a notable financial and health risk during accidents where various hazards can help unleash the fission products outside the fuel clad. A nuclear reactor must address this source term head on. It must protect the powerplant investment and surrounding community from the radioactivity. To do so, it must contain fission products and radiation by remaining at safe temperatures across the condition space including operations, beyond design basis accidents (BDBA), and disposal.
We must not conflate the existence of the fission products with potential consequence. A source term can only have a large consequence if there is a driving force to energize and disperse the fission products. In a nuclear reactor, that energetic driving force is usually the decay heat power, rapid power increases during a reactivity accident, unexpected loss of cooling, or a chemical energy release – but can be any of the hazards shown in Table 4. Removing the driving forces and hazards removes the consequence. This is as far as one can go to reduce consequence while still generating neutrons and fission products. All fission reactors have a source term, but not all reactors have an energetic driving force to turn those fission products into a consequence. A nuclear corolla should aim to reduce energetic driving force and contributing hazards that can create a consequence from fission products. Achieving this in a cost-effective manner, has remained elusive.
Risk = Probabilities • Consequences
Aversion to nuclear deployment is more subtle than just an overreaction or an overinflated risk perception. While deaths per TWh attributed to nuclear power, including those from black swan accidents, are on par with renewables,[1] and while reactor accident event frequency has been reduced from 0.01 in the 1970s to 0.003 events per plant per year worldwide through industry reform and learning, we have simultaneously seen the less frequent but extremely high-cost accidents every ~6,000 reactor-years. There have been 24,000 commercial reactor years and 4 of the commercial ones have undergone meltdowns. These accidents pose a low probability but high consequence financial risk relative to alternative technologies.[2]
The majority of operating nuclear power plants are statistically safe on death per TWh metrics because the death statistics are a shallow way of measuring consequences, and the statistics are theoretically diluted across very large populations. But the local adverse effects of an accident to the utility, asset owners, and customers are concentrated and crushing. Coal and fossil fuels are statistically less safe by the same metric, but any climate change and health effects are diluted worldwide in a real and practical way. The reality is that there are cost competitive alternatives to nuclear energy, and these systems are not able to fail catastrophically like a nuclear reactor because they do not have fission products onsite. Why expose oneself to the even small probabilities of extreme consequences when there are alternatives?
Ordinarily, comparisons are made on the deaths per energy unit delivered, as in the below figure. But the public is more concerned with significant hardship than with simple deaths. A better metric than death rates is a death equivalent consequence like displacement or financial loss. It appears likely that excess death rates from accidents like TMI and Fukushima are too low or too difficult to measure over the decades. But the financial cost of a nuclear cleanup and forced population displacements of the region surrounding the power plant can be considered equivalent to deaths. Forced displacement, prolonged financial hardship, loss of land are equivalent to death. Financial losses diluted over large populations can be converted to deaths even without a single actual death.

The below equation represents the concept of risk as the probability of an event multiplied by its consequence. [5] added a power of to the consequence to incorporate the idea that perception of consequence does not always match the real consequence. While we can assume perception matches reality, we suspect that the public is an adherent to Murphy’s Law, and increases to compensate lower theoretical probabilities from engineers, a kind of hedge against the word of those who stand to benefit most and often have no skin in the game. With , risk is often perceived simply as the potential consequence.
\[ Risk=PC^\alpha \]
We have seen nuclear accidents play out over the last 60 years, with a reported probability of about 1/100 reactor failures with severe local consequences, perhaps double if including close calls, and extra dangers associated with spent fuel pools. This comes out to about 1/6,000 probability of a severe nuclear accident per reactor year. The consequences of these accidents have proven substantially different from other sources of energy. In particular, the health consequences have been limited or comparable to other sources of energy, while the financial consequences have been immediate and long lasting, staggeringly high and concentrated to the regions around the reactor.
Direct effects of nuclear accidents have historically been geographically constrained to within tens of kilometers and at most a hundred kilometers if the weather is particularly unfavorable. There is little reason to believe nuclear accidents can directly have global effects because radioactivity is massively diluted with distance and travel time, and weather is a comparatively slow phenomenon relative to the most relevant radioisotopes. For nuclear, the risk of serious financial or health disaster is a local matter with local consequence.
Fossil fuels damage at both local and global scales. The pollution around fossil fuel plants reliably increases respiratory diseases and cancers. The production of greenhouse gases is a global effect, but its financial and health impact have not yet managed to justify a full decarbonization at the expense of slower economic development. Financial losses due to changes in climate, should the predictions ever materialize, may be recouped through newly available and useful land at higher latitudes. For fossils, in the worst case of power plant destruction, the utility or insurer will lose the value of the power plant and a new one can be built. There are no century-long cleanup efforts for fossil power plant accidents. There are no bankruptcies at the city, company, or country level. But the group risk is higher because of the health consequences of polluting the atmosphere and perhaps some climate change effects could materialize at some point.
Financial consequences of fossil fuel use are not clearly measurable owing to the great dilution, long time frames, and guesswork involved in its estimation. Fossil fuel health consequences are globally diluted. The probability of death-like consequences from fossil fuel pollutants is in the USA, [6] equally applied to the entire population.
From a global planner perspective, nuclear makes sense if the nuclear risk is less than the fossil risk. The risk is computed for each course of action below. The risk of each choice is the product of the population exposed, the probability of consequence, and the consequence itself which is a death or death equivalent consequence. For nuclear the reactor accident probability multiplied by the local population is considered. For fossils, the probability of death from emissions multiplied by the total population is considered.
\[ P_Nn_NC_{death,eq}<P_Fn_FC_{death,eq} \]
We can drop the , the consequence, since it's on both sides. Compared to fossils, nuclear doesn't have to have as low an event probability because its accidents can be locally confined. That is . The risk averse global planner chooses nuclear if the following inequality is met.
\[ P_N\frac{n_N}{n_F}<P_F \]
But for the local planner and local population, where nuclear only makes sense if the nuclear event probability is lower than the fossil event probability.
\[ P_N<P_F \]
The problem is that and are perceived as roughly equivalent and could well be about the same in reality. Historically, we know that most of existing reactors fail at a rate of about 1/6000 per year, with extreme costs, concentrated on the local populations and countries of operation. With this history and this comparison, a rational, local population sees roughly equivalent risk from either choice. But more likely, the local population perceives , in deference to Murphy’s Law. Fossil exposures are mostly conventional deaths and not easily traceable to fossils – it's difficult to blame fossil fuels for the premature cancer and respiratory deaths of a 60-80 year old, especially considering that fossil burning has been the norm for three centuries. And one can blame the whole fossil fuel industry, not any single fossil fuel emitter. Nuclear accident consequences have a clear source. It’s easy to connect the financial and death equivalent consequences related to a specific nuclear reactor accident.
The local population also has no influence over the fossil fuel emitters. Their exposure to fossil risks is not affected by their local choice of nuclear or fossil energy. For the local population, choosing nuclear only adds to the fossil risk. On the other hand, the global planner is willing to put some populations at elevated risk to achieve lower overall risk. While globalist autocratic societies are on the rise, we hope self-determination by local people remains the way of the west. With this understanding, massive nuclear deployments in democratically run countries requires a new approach to risk reduction – one based on a deterministic approach that better approximates the public’s respect for Murphy’s Law.
Risk Reduction Via Lower Probabilities
The typical design approach for nuclear reactors has been to avoid accidents at all costs because the fuel can fail catastrophically in such events, due to melting or rupture. As a result, nuclear powerplants have become overengineered behemoths with a complex web of safety systems aimed at reducing the probability that accidents occur and trying to contain them when they do occur. Costs balloon rapidly, in large part due to paperwork but also due to quality control, material costs, and inefficient construction.[7] Accidents are yet to happen in GENIII and III+ reactors but GENI and II designs’ accidents have resulted in unexpected fission product release and loss of public trust that continues to limit the industry’s growth in certain regions of the world. To become viable decarbonization tools, nuclear reactors must simultaneously eliminate black-swan risk and reduce costs.
Reducing reactor accident rates is expensive in large and traditional nuclear reactors. For new reactors and new components including passive safety systems, the probabilities are educated guesses that remain to be tested in time. During licensing, reactor designers make a safety case by using Probabilistic Risk Assessment (PRA). They analyze event trees that can lead to catastrophic accidents and assign probabilities to the events to come up with “core-damage frequencies.” PRA emerged as a tool to figure out where the engineering design should be focused to reduce risk most efficiently. Worst case high consequence accidents like complete Loss of Coolant Accidents (LOCA) are not likely and somewhat impossible to deal with, so the focus shifted towards dealing with lower consequence but higher probability accidents like partial LOCAs which would have more impact on the overall risk. This is a sensible way to lower the overall risk as economically as possible but still leaves reactors exposed to worst case black swan accidents that could occur despite very low theorized probabilities.[8]
But even very low probabilities lead to reasonably high chances of failure at very high deployments. This is why the operating reactors rely on containment for both predicted low probability events and unknown events, the containment limits the radioactive release and exposure of it to humans. Those failures, rare as they are, despite the ability of containment to reduce off-site dose consequences, still lead to a global slump in financial and political support – the nuclear resets. The black swan risk is always there. Regulators, bankers, and the public are ready to slow down nuclear adoption, throttle financing and approvals, and heap on a few more regulations. Does the public care about these estimated PRAs considering the history of nuclear accidents and prior erroneous claims? No matter how low the estimated probability or how much extra CAPEX and OPEX goes into lowering the probability, the public is likely to continue to respect Murphy’s Law and will pick amongst the many available alternatives.
Risk Reduction Via Lower Consequences
Minimizing probabilities is a fool’s game that can only increase costs because the underlying problem is not solved. We should avoid building and operating reactors in such a way that they can melt during inconvenient conditions. The alternative approach to risk reduction is to lower consequences and embrace accidents through passive safety systems as almost all new reactors world-wide have limited to full reliance on passive safety. In CA-HTGR, the probabilities in PRA are generally set to 1 and the safety analysis becomes more deterministic. For CA-HTGR, consequences of accidents are negligible because of the inherent safety mechanisms that can deal with the decay heat. This means that reactor designs should not have to include elaborate systems to lower the theoretical probability of accidents from happening.
This deterministic, Murphy’s Law approach accepts that worst case accidents will happen at some point, commonly refer to as the maximum credible accident, and then designs the reactor and fuel so that the worst-case accident has low and if not zero nuclear related consequences. This is achieved by reducing the hazards and driving forces that can turn fission products into a consequence. More practically, this can be done by designing the reactor to passively remain at safe temperatures in worst case accident conditions including simultaneous Beyond Design Basis Accidents (BDBA). The ability to safely dissipate the reactor’s energy can be relegated to passive physical mechanisms rather than active systems.
No one doubts that a nuclear reactor can be designed this way. But the idea that it can be done at competitive costs remains in question. The reduction in consequence has far reaching implications beyond potential public acceptance. Using passive heat dissipation systems, the reactor can be simplified with fewer safety systems and parts. Rather than trying to avoid accidents through perfect operations and safety systems, these reactors would rely on physical material properties to withstand accidents rather than external or active systems. This means worst case accidents can happen without fission product release – a radical shift from today’s reactors in which mechanical cooling capability is required to avoid meltdown. Without conventional meltdown risk, these reactors may be treated like non-nuclear industrial sites with a corresponding reevaluation of costs and applications. The nuclear corolla takes this approach aiming for a cost profile of CCNG if the nuclear regulation treats it as such.
[1] Ritchiem and Roser, “Modern Renewables and Nuclear Energy Are Not Only Safer but Also Cleaner than Fossil Fuels.”
[2] Wheatley, Sovacool, and Sornette, “Reassessing the Safety of Nuclear Power.”
[3] Wheatley, Sovacool, and Sornette.
[4] Ritchiem and Roser, “Modern Renewables and Nuclear Energy Are Not Only Safer but Also Cleaner than Fossil Fuels.”
[5] Weinberg, “Science and Trans-Science.”
[6] Ritchiem and Roser, “Modern Renewables and Nuclear Energy Are Not Only Safer but Also Cleaner than Fossil Fuels.”
[7] Eash-gates, Kavlak, and Mcnerney, “Sources of Cost Overrun in Nuclear Power Plant Construction Call for a New Approach to Reactor Engineering Design.”
[8] Zinkle et al., “Accident Tolerant Fuels for LWRs: A Perspective.”